I started working on arc-sites for OpenVoronoi. The first things required are an in_region(p)
predicate and an apex_point(p)
function. It is best to rapidly try out and visualize things in python/VTK first, before committing to slower coding and design in c++.
in_region(p)
returns true if point p
is inside the cone-of-influence of the arc site. Here the arc is defined by its start-point p1, end-point p2, center c, and a direction flag cw for indicating cw or ccw direction. This code will only work for arcs smaller than 180 degrees.
def arc_in_region(p1,p2,c,cw,p):
if cw:
return p.is_right(c,p1) and (not p.is_right(c,p2))
else:
return (not p.is_right(c,p1)) and p.is_right(c,p2) |
def arc_in_region(p1,p2,c,cw,p):
if cw:
return p.is_right(c,p1) and (not p.is_right(c,p2))
else:
return (not p.is_right(c,p1)) and p.is_right(c,p2)
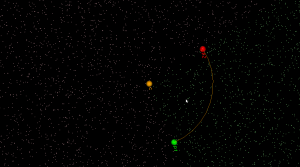
Here randomly chosen points are shown green if they are in-region and pink if they are not.
apex_point(p)
returns the closest point to p
on the arc. When p
is not in the cone-of-influence either the start- or end-point of the arc is returned. This is useful in OpenVoronoi for calculating the minimum distance from p
to any point on the arc-site, since this is given by (p-apex_point(p)).norm()
.
def closer_endpoint(p1,p2,p):
if (p1-p).norm() < (p2-p).norm():
return p1
else:
return p2
def projection_point(p1,p2,c1,cw,p):
if p==c1:
return p1
else:
n = (p-c1)
n.normalize()
return c1 + (p1-c1).norm()*n
def apex_point(p1,p2,c1,cw,p):
if arc_in_region(p1,p2,c1,cw,p):
return projection_point(p1,p2,c1,cw,p)
else:
return closer_endpoint(p1,p2,p) |
def closer_endpoint(p1,p2,p):
if (p1-p).norm() < (p2-p).norm():
return p1
else:
return p2
def projection_point(p1,p2,c1,cw,p):
if p==c1:
return p1
else:
n = (p-c1)
n.normalize()
return c1 + (p1-c1).norm()*n
def apex_point(p1,p2,c1,cw,p):
if arc_in_region(p1,p2,c1,cw,p):
return projection_point(p1,p2,c1,cw,p)
else:
return closer_endpoint(p1,p2,p)
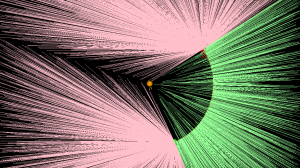
Here a line from a randomly chosen point p
to its apex_point(p)
has been drawn. Either the start- or end-point of the arc is the closest point to out-of-region points (pink), while a radially projected point on the arc-site is closest to in-region points (green).
The next thing required are working edge-parametrizations for the new type of voronoi-edges that will occur when we have arc-sites (arc/point, arc/line, and arc/arc).